Probing the dynamic forces that move 37 trillion cells in the human body
Gregory M. Alushin, head of Rockefeller’s Laboratory of Structural Biophysics and Mechanobiology, doesn’t think about his career in science as a predestined calling as much as the result of accumulated experiences.
“It makes a good story to say that you’re very intentional,” he says, “but I think there are a lot of circumstances that determine our path.”
The same can be said about the approximately 37 trillion cells that make up the human body. Each has its own identity and function, shaped in part by the external forces it encountered throughout its life—including mechanical forces, like the incessant push-and-pull from neighboring cells. Alushin studies how these little-understood physical dynamics act on the cell’s cytoskeleton, an internal network of protein filaments that constantly reconfigures itself to help the cell move, change shape, or ferry molecules from one cell compartment to another.
Beyond revealing fundamental truths about this molecular machinery, Alushin’s work has implications for understanding how organisms grow and develop, and how the biomechanical dynamics that some cancers use to metastasize from one organ to another could be used against them.
From writer to biochemist
Growing up as an only child in University Park, Maryland, a small commuter town outside Washington D.C., he wanted to be a writer. He was first drawn to fiction, rereading James Joyce’s Ulysses once a year, and then got interested in journalism. His mother taught French in a public school and his father was an environmental lawyer for the U.S. Environmental Protection Agency (EPA).
Alushin’s writerly ambitions gave way to a different passion when he took an organic chemistry class in his first undergraduate year at Columbia University. “I got excited about the idea of the world of molecules being this different, mysterious realm,” he says. “For me, it was unexplored territory.”
By the time he was in a PhD program at Berkeley, he’d dived deep into biochemistry and structural biology, studying how ribosomes and other higher-order assemblies take shape. But trying to capture the conformations of proteins using X-ray crystallography, which was the standard methodology back then, frustrated him. The first step in studying a protein’s shape was to coax it into forming a crystal—and so many proteins refused to crystallize, making success “a bit too random for my taste,” he says.
He switched to an emerging method in which frozen proteins were visualized with an electron microscope—and while it improved his batting average, it too wasn’t easy. “Back then, electron microscopy methods to study structures were in their infancy, so one of the things we had to do was to take pictures of proteins using photographic film,” he says.
He spent many nights alone in a pitch-black lab, utterly silent, utterly still, as he attempted to record protein structures on film, not daring to breathe lest the vibrating air make the image blurry. But he liked the quiet and the solitude of these red-eye shifts, which gave him the chance to make images by night and share them with colleagues by day.
And his efforts ultimately paid off, allowing Alushin and his colleagues to produce the highest-resolution images of microtubules—cytoskeletal filaments that give a cell its shape—that had ever been achieved at the time.
Let’s get physical
As a researcher with his own lab at the National Institutes of Health, he then became fascinated with the idea that cells have a sense of touch that they use to perceive their surroundings. He shifted his focus from microtubules to actin filaments, another key part of the cytoskeleton made up of long polymers of proteins linked end-to-end. Actin filaments power cellular movement, bind with hundreds of different cellular components, and serve as tracks for molecular motor proteins.
They also intrigued him because they seemed to merge a classical way to think about biology—as a system of genes and proteins—with the lesser understood concept of mechanobiology, “occupying an existential gray area between the microscopic chemical and the macroscopic physical worlds,” Alushin says. “I wondered: Can we learn something new about how actin filaments make use of this duality of their nature?”
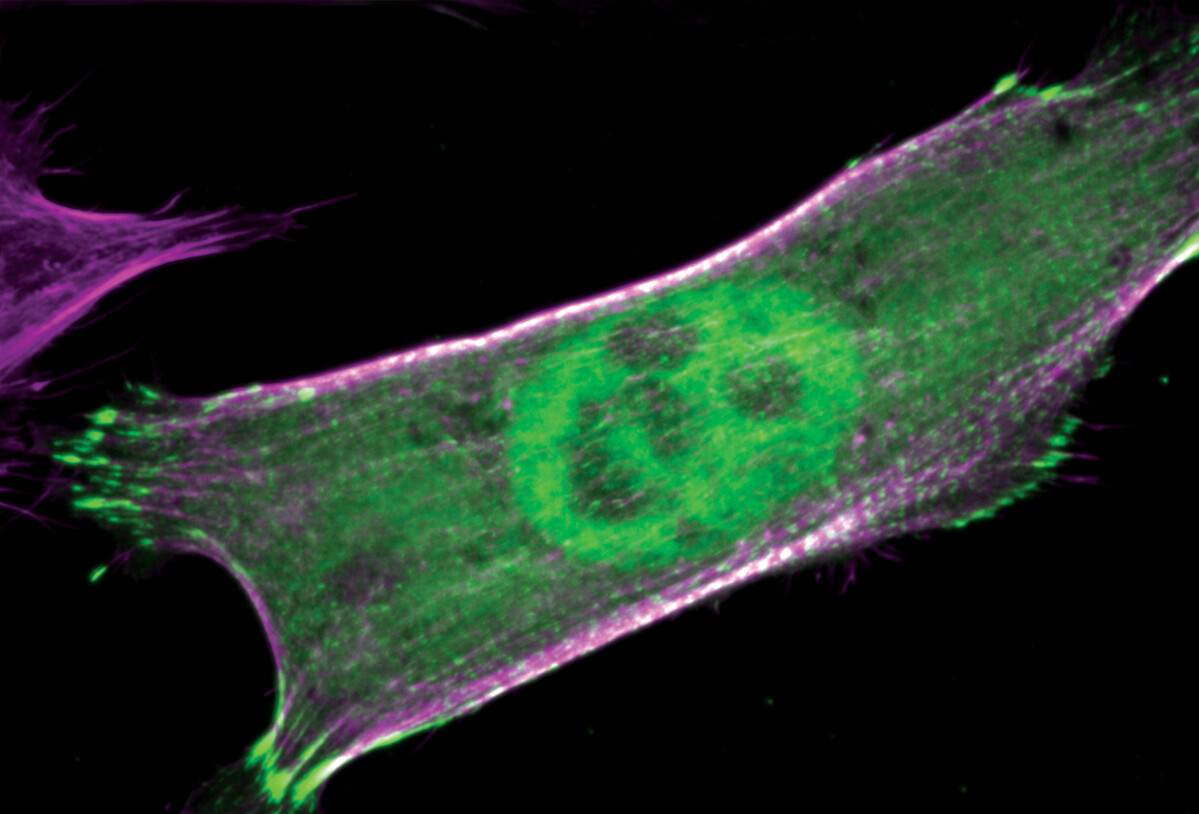
Actin fibers and nucleus in a cell (Alushin Lab)
Actin filaments remain his focus today at Rockefeller, where he has headed his lab since 2017. While we know these wiggly proteins move the cell around, detect forces in the cell’s surroundings, and apply force, their mechanobiology remains largely undiscovered. How do their cellular functions relate to their bending and flexing, crisscrossing each other, and having tugs of war?
He uses a variety of biochemical and biophysical methods to reveal the molecular processes that underlie how a cell senses and responds to its physical context. And thanks to today’s technology being much more advanced than in his graduate-school days, Alushin no longer needs to hold his breath in the dark to get a good image. Instead, he books time in the Cryo-Electron Microscopy Resource Center, where the highly sophisticated instruments produce images that allow him to visualize the 3D structures of molecules and macromolecular complexes.
Alushin’s team recently captured the first images of actin filaments as they experienced forces that altered their structure. “That was very satisfying,” he says, “because it shows we can explore the intersection between protein biochemistry and physical force, a new concept that might deepen our understanding of what a protein can do.”
Fundamental—but exploratory
His research is highly relevant for cancer research because many of the processes a cancer cell relies on to thrive—like hogging resources, dividing uncontrollably, and spreading to new organs—require it to move.
Moreover, cancer cells move differently from normal cells. “They use different physical mechanisms for reorganizing their cytoskeleton, and they undergo very dramatic shape changes,” Alushin says. “And interestingly, they rely more on nonspecific forces like friction and pressure to get from A to B than healthy cells do.”
He suspects that if cancer cells use unique physical dynamics to move, those same properties could prove to be their Achilles heel. If we could just understand how to target cells that move in a cancer-like fashion, it might be possible to eradicate tumors while causing minimal harm to healthy cells and tissues.
Collaborating with colleagues at Memorial Sloan Kettering Cancer Center, Alushin’s lab is also using cryo-EM to study the biomechanics of how the immune system’s T cells engage with cancer cells. The team has created a system for stimulating T cell activation using cryo-EM substrates—little gold discs that look like glitter—so that they can record T cells in action and observe the workings of their actin filaments. The scientists hope that such studies could one day translate into the development of immunotherapy drugs that help stimulate cancer-fighting T cells.
However, Alushin is under no illusion that his discoveries will make it from bench to the bedside anytime soon. After all, he’s doing basic research on the cutting edge of a nascent field whose medical implications are still being established.
And that’s fine with him. “My personal satisfaction comes from figuring out the fundamental mechanisms. I’ve just always enjoyed the intellectual beauty of that,” he says. “Science is as much an art project for me as something focused on producing practical benefits.”
Sharp eyes and insights
A 2021 winner of the Rockefeller University Distinguished Teaching Award, Alushin encourages the students and postdocs in his lab to take a similarly artistic approach. “There’s definitely a creative component to designing a good protocol so that you get clean, beautiful results,” he says.
A sharp eye for interesting details is also essential, he notes. “That’s one of the things that really distinguishes a great scientist in my field,” he says. “It’s visual work. To land on something that’s really exciting, you need to be able to look at busy, complicated images and say, ‘Oh, that’s the cool thing. Let’s look into it.’”
“I love mentoring graduate students,” he enthuses. “It’s such an exciting career stage. People usually know enough to make independent progress on their research, but they’re also openminded, not yet set in their ways. It’s a great time to do bold, pie-in-the sky work.”
As for his own high hopes, he says, “my fantasy is that we will discover something totally different about how proteins work in the context of force. That would be a really important discovery, because it would open up new ways of thinking about how molecules operate in biology.”