Gaby Maimon, who studies sophisticated brain functions in fruit flies, is promoted to associate professor
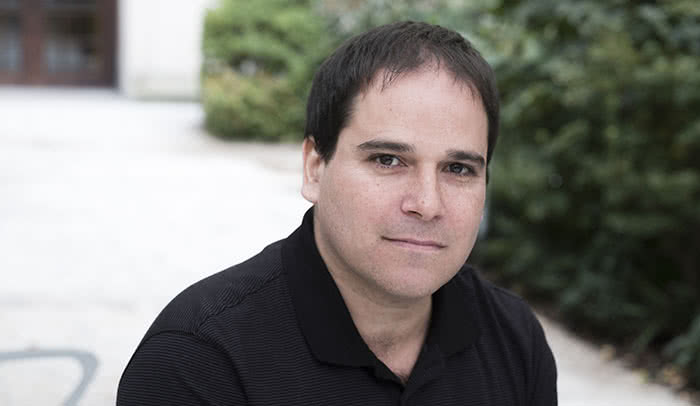
Gaby Maimon uses the small brains of fruit flies to ask the types of questions that are much more difficult to address in more complex mammalian brains.
Neuroscientist Gaby Maimon, who heads the Laboratory of Integrative Brain Function, will become an associate professor as of January 1, 2017. His research program explores how the brain performs calculations to estimate values like angles and time, and is based on the idea that fruit flies, his research subjects, have more in common with humans than one might think.
With about 100,000 neurons, the fly brain is only a fraction as big as the human brain, which has over 80 billion neurons, and is considerably less complex. Even so, Maimon’s lab is demonstrating that the fly brain is capable of performing many of the same internal calculations.
“These sorts of computations form the basis of cognition, however little is known about how any brain carries them out,” Maimon says. “Our work so far suggests flies have a great deal more to tell us about higher brain function than has been appreciated.”
“Gaby is a highly original scientist, and his work is opening up a new field of inquiry within neuroscience, one that offers a fresh approach to fundamental questions,” says Richard P. Lifton, the university’s president. “His research has the potential to further our understanding of the human brain and to help explain, at a basic level, how nature has constructed systems for making complex decisions about behavior.”
The Board of Trustees approved his promotion on October 19.
Tiny brains, complex behavior
The brains of mammals, including humans and rodents, are capable of sophisticated calculations based on variables they store and update, such as distances, short intervals of time, or the value of various food sources. There has long been evidence that at least certain insects are capable of similar processes. Ants, for instance, can find their way back to their nests, and honeybees can communicate the location and quality of flowers or potential new hive sites.
For a scientist looking to better understand these calculations, fruit flies offer significant advantages as study subjects: their genetics and development are well understood, and sophisticated tools for studying their neurons already exist. Maimon’s goal is to use their more tractable small brains to ask the types of questions that are currently much more difficult to address in more complex mammalian brains.
To do so, however, he faced significant challenges. “No one studied these processes in flies for two reasons. First, it wasn’t clear that the fly brain was sophisticated enough to even keep track of short time intervals, or angles used for navigation, or other quantitative internal variables,” Maimon says. “Second, to find evidence of these, you need to look into the tiny fly brain, which is only a fraction of the width of the head of a pin, while the animal is actively engaging in a behavior.”
As a postdoc at The California Institute of Technology in the late 2000s, he developed the first system for monitoring and even altering electrical activity within the fruit fly’s brain while it performs a behavior such as moving about. To accomplish this, he developed a micro-scale platform to which he could attach flies in such a way they could still flap their wings or walk on the tiny equivalent of a treadmill. The setup allows Maimon to offer his subjects stimuli, such as visual images projected on an LED display or puffs of air, and record the insects’ responses.
Doing the math
When he established his lab at Rockefeller in 2011, Maimon set out to prove flies could be used to study at least rudimentary aspects of cognitive processes. His lab has recently identified a neural circuit that explains how the brain can rotate an internal compass signal, only recently discovered to exist in insects, even when the fly is in the dark with no visual cues to fall back on. Scientists suspect rodents might use a similar system to accomplish the same computation.
The lab found another potential parallel between the fly brain and that of mammals when studying the brain’s ability to compensate for the type of motion the visual system experiences due to the animal’s own actions. Scientists have long suspected that every time the body moves, the motor system alerts the visual system so it can process the motion caused by self-generated movements differently than motion caused by events in the outside world.
The notion that visual systems might separate motion in this way arose from behavioral experiments in flies in the 1950s. Experiments in Maimon’s lab proved that the fly brain makes these computations and demonstrated how it does so. They discovered motion-canceling signals in the fly visual system, which are carefully calibrated to eliminate the predicted visual stimulus caused by flight turns, while allowing the system to remain sensitive to unexpected stimuli.
Other studies have examined how flies estimate short time intervals, and how they evaluate the relative quality of potential sites to lay their eggs.
Looking for a template
Among other ongoing projects, Maimon now plans to identify the cells that bring the motor-related canceling signals to the visual system, and explore whether this system is relevant to human eye movements. His lab will also be investigating the role of a part of the fly brain known as the central complex in spatial navigation and other internal computations.
“Many animals, including humans, perform quantitative calculations, yet we don’t have a detailed understanding of the mechanics by which any brain does so,” he says. “Our big-picture goal is to provide detailed examples of how a brain can store numbers and use them to influence behavior.”