Hundreds of new drug targets to combat tuberculosis
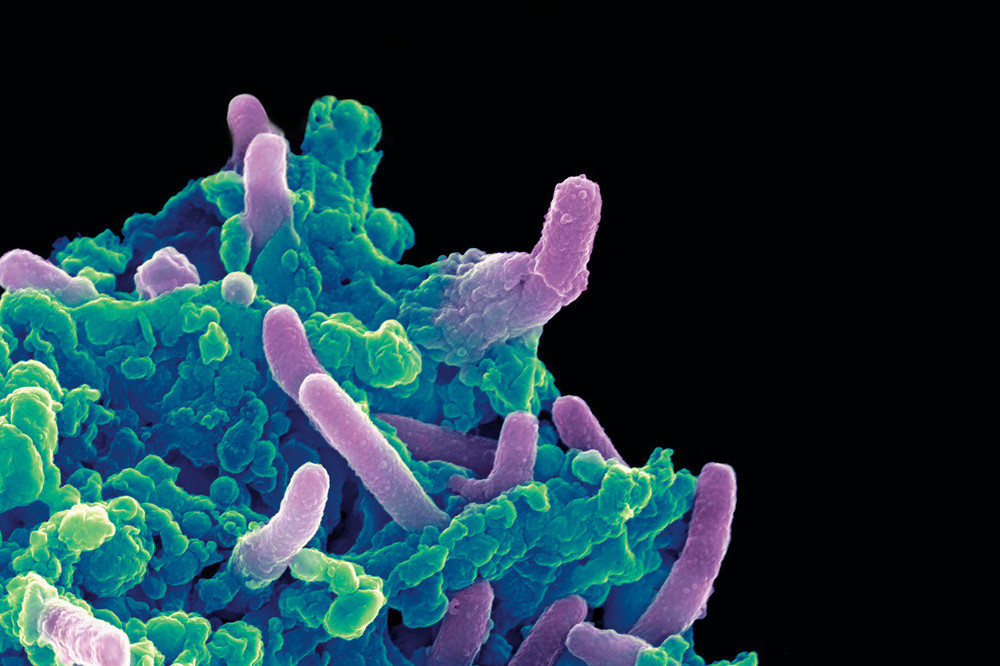
Colored scanning electron micrograph of Mycobacterium tuberculosis bacteria (purple) infecting a macrophage.
Tuberculosis is a stubborn disease, born of yet more stubborn microbes. While many bacterial infections resolve within days of starting antibiotics, tuberculosis often refuses to budge for around six months and, in some cases, may never release its vise grip on the human body. It claimed 1.5 million lives in 2020, second only to COVID-19 among infectious diseases.
Now, a new study in Nature Microbiology maps the methods by which Mycobacterium tuberculosis (Mtb) bacteria shrug off antibiotics, revealing hundreds of drug targets that could strip this pathogen of its notorious resistances. The scientists also identified a class of existing antibiotics that may be particularly effective against one prominent strain in Southeast Asia.
“Examining how current drugs affect bacterial physiology, and how the bacterium subverts this, is part of our long-term goal of developing better treatment combinations,” says Rockefeller’s Jeremy Rock, head of the Laboratory of Host-Pathogen Biology. “This study is the tip of the spear getting us into that realm.”
A genome-wide view of Mtb
The researchers started out with a simple question. “We just wanted to know all of the genes involved in Mtb’s resistance to different antibiotics,” says Nick Poulton, a graduate student in Rock’s lab and coauthor on the study. The team developed a platform based on the CRISPR gene knockdown tool that scoured the Mtb genome, pitting the bacteria against common antibiotics to quantify how the absence or presence of each gene impacted the efficacy of existing drugs.
“This allowed us to study essentially every gene in the Mtb genome in a single pooled library, and we were able to inhibit the expression of specific genes without making permanent changes to the bacterial genome,” Poulton says. They ultimately identified 1,373 genes that, when silenced, rendered Mtb vulnerable to antibiotics and another 775 genes that had the opposite effect—when the latter genes were silenced, the bacteria developed stronger resistance.
Two genes in particular, mtrA and mtrB, rendered Mtb highly susceptible to existing therapies. These genes are responsible for maintaining the bacterium’s protective coating, and likely play a role in Mtb’s natural ability to resist antibiotics. The researchers hope that future therapies could boost the effectiveness of current antibiotics by thwarting the genes’ function.
“When you inhibit these genes, a number of bacterial-cell processes goes awry,” says Shuqi Li, a former postdoctoral fellow in the Rock lab and coauthor of the study. “This renders Mtb sensitive to many drugs that would otherwise have been less effective.”
Rock, Poulton, Li, and colleagues also identified several previously unknown mechanisms by which mutations in the bacA gene contribute to drug resistance. “We’re seeing treatment failure all over the place, and we wanted to better understand the genetic mechanisms that may be leading to resistance,” Poulton says. “The bacA mutations that we discovered may be a unappreciated source for resistance to second-line drugs, and should be monitored more closely in the clinic.”
Optimizing new antibiotics
After these initial findings, the team turned their attention to linezolid, a recently-approved antibiotic that is highly potent against Mtb, but can cause serious side-effects. Rock and colleagues wondered whether their dataset might be able to inform ongoing efforts around the world to optimize linezolid so that it remains effective even in lower, less toxic, doses.
Indeed, they found two genes that, when inhibited, rendered Mtb more sensitive to linezolid by acting on ribosomes, the molecular machines that translate a bacterium’s RNA into protein. Since linezolid works by gumming up bacterial ribosomes, the team suspects that these genes fight back against the drug and contribute to resistance by rebooting frozen protein machines.
When the team silenced both genes, non-resistant Mtb became twelve times more sensitive to linezolid. Their results in drug-resistant Mtb were even more impressive: Knocking down both genes appeared to undo antibiotic resistance altogether, turning resistant Mtb back into normal Mtb. “If you could find drugs that inhibit these two pathways, it might theoretically be possible to fully restore linezolid susceptibility back to wild type levels,” Poulton says.
Even in non-resistant strains, inhibiting those two genes could boost linezolid efficacy enough to allow clinicians to administer lower doses, potentially reducing the negative side-effects.
Dusting off shelved drugs
In the future, these findings could have considerable implications for drug developers seeking to address the growing problem of antibiotic-resistant tuberculosis. Moreover, the Rock lab made an additional discovery that might save lives even in the short-term.
The team noticed that one Mtb strain, responsible for half a million tuberculosis cases each year in Southeast Asia, had naturally acquired an odd mutation in a gene known as whiB7. They suspect that the mutation arose about 900 years ago, and it’s unclear what advantage, if any, it conveyed. What is clear is that this mutation renders the bacterium extremely vulnerable to a class of safe, well-tolerated, and FDA-approved antibiotics, known as macrolides.
Although macrolides are frequently prescribed to treat other infections, they have not historically been used in the treatment of TB. “Our research in vitro and in mice suggests that this lineage may be hyper-susceptible to macrolide drugs,” Rock says.