Revealing how an ancient genetic invader inhabits our DNA
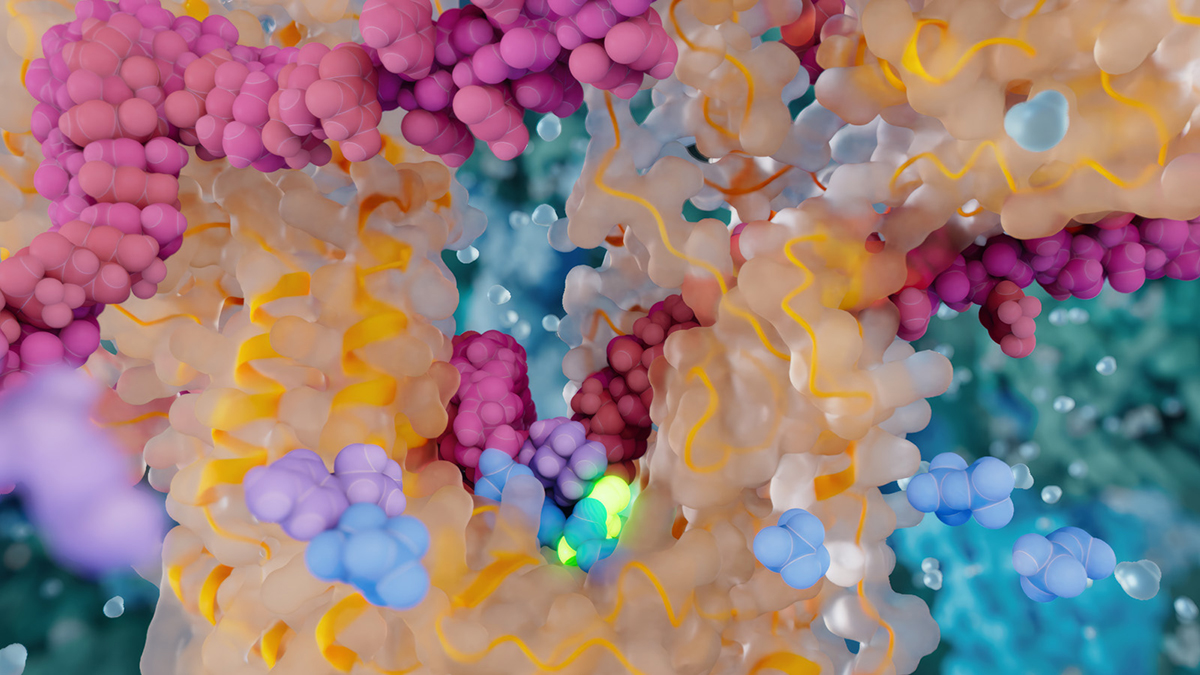
The active site of the ORF2p reverse transcriptase (Visual Science)
Billions of years ago, as primitive lifeforms were becoming more complex, a selfish genetic component became a sort of genome colonizer. Using a copy-and-paste mechanism, this pernicious bit of code replicated and inserted itself again and again into a variety of genomes. Over time, all eukaryotic organisms inherited the code—including us. In fact, this ancient genetic element wrote about one-third of the human genome—and was considered junk DNA until relatively recently.
This genetic component is known as LINE-1, and its aggressive intrusion into the genome can wreak havoc, leading to disease-causing mutations. A key protein called ORF2p enables its success—meaning understanding ORF2p’s structure and mechanics could illuminate new potential therapeutic targets for a variety of diseases.
Now, in collaboration with more than a dozen academic and industry groups, Rockefeller scientists have rendered the protein’s core structure in high-resolution for the first time, revealing a host of new insights about LINE-1’s key disease-causing mechanisms. The results were published in Nature.
“The work will facilitate rational drug design targeting LINE-1 and may lead to novel therapies and strategies to combat cancer, autoimmune disease, neurodegeneration, and other diseases of aging,” says senior author John LaCava, a research associate professor at The Rockefeller University.
Evolutionary mates
LINE-1 is a retrotransposon, a kind of mobile genetic code that translates RNA back into DNA while replicating and writing itself into different places throughout an organism’s genome. There are different kinds of retrotransposons, including endogenous retroviruses (ERVs), which resemble HIV and Hepatitis B (HBV).
LINE-1’s origin is unclear, but it has an evolutionary connection to group II introns, a class of ancient mobile elements dating back about 2.5 billion years. Retrotransposons like LINE-1 have been evolving with their host organisms for 1 to 2 billion years.
“It’s an ongoing battle between LINE-1 trying to insert itself and the host protecting its own genome,” says co-first author Trevor van Eeuwen, a postdoctoral fellow in Rockefeller’s Laboratory of Cellular and Structural Biology.
Millions of genetic fragments derived from LINE-1 are found in our cells. The vast majority are inactive evolutionary relics, evidence of failed attempts to hijack the replication machinery. But about 100 LINE-1s are operational—and usually not helpful. One protein produced by LINE-1, known as ORF1p, is churned out by cancer cells, as a recent study by LaCava, Michael P. Rout, and their collaborators described.
LaCava and Martin Taylor, of Massachusetts General Hospital and Harvard Medical School, have collaboratively studied LINE-1 and its proteins for more than a decade, but because ORF2p expresses so lowly and infrequently, it has remained poorly understood. “LINE-1 has been so difficult to study because it has very weird features,” LaCava says. “For instance, it has an unusual replication cycle and the ORF2p protein that no one’s been able to capture. But Marty and I eventually reached a place where our research on it was mature enough so that we could begin to study its structure.”
Taylor made key advancements in purifying the full-length ORF2p as well as a shorter “core” version that facilitates L1 replication; these advances facilitated the breakthroughs that followed.
Jack-of-all-trades
Using a combination of x-ray crystallography and cryo-EM, the research team discovered two novel folded domains within ORF2p’s core that contribute to LINE-1’s ability to make copies of itself.
ORF2p has structural adaptations uniquely suited for these endeavors, says van Eeuwen. It’s a sort of jack-of-all-trades protein, capable of handling everything from replication to insertion. But while most viruses need potentially hundreds of reverse transcriptase proteins to replicate, ORF2p does it all.
Yet when LINE-1 is activated in the cytoplasm, “it acts like a viral mimic. It creates RNA:DNA hybrids that look like a viral infection when they’re sensed,” van Eeuwen notes. This viral mimicry suggests a possible solution to the puzzle of how ORF2p activates the innate immune system, contributing to autoimmune disease and other conditions. Their research found that interactions with genetic material in the cytoplasm activate the cGAS/STING antiviral pathway. In turn, that pathway causes cells to produce interferons, stimulating the immune system and leading to inflammation, in a manner analogous to what happens during an infection by a virus.
“Its main function appears to be to proliferate copies of itself, and as LINE-1 moves sequences around, there’s a chance those sequences could break a gene,” he says. “But there’s also a chance they could create new genetic elements or novel functionalities that are beneficial to the host.”
The path ahead
In the future, the researchers will seek to solve the two newly discovered core domains and understand their functions. In the meantime, “our structural elucidation of ORF2p lays the groundwork for future studies needed to dissect and improve our understanding of the LINE-1 insertion mechanism, its evolution, and its roles in disease,” van Eeuwen says.
They also want to explore the potential clinical applications of their findings. Because there is a kinship between retrotransposons and retroviruses, in the current study they tested treatments for the retroviruses HIV and HBV to see if they would inhibit LINE-1. They did not, suggesting that the design of therapeutics will have to be tailored to LINE-1’s unique characteristics.
“The work opens the door to rational drug design of better LINE-1 inhibitors, and we hope these will lead to clinical trials soon,” LaCava says.
And, as Rout adds, “this study also underscores the potential of integrating multiple kinds of data—and multiple labs’ expertise—to solve fundamental biomedical questions.”
This research project was an international collaboration. In addition to scientists from Rockefeller, crucial contributions were made by ROME Therapeutics, the University Medical Center Groningen (J. LaCava lab), MSKCC (B. Greenbaum lab), Rutgers (E. Arnold lab), University of Alberta (M. Götte lab), Dana Farber (K. Burns lab), UCSF (A. Sali lab), MPI Tübingen (O. Weichenreider lab), and UT Arlington (S. Christensen).