Intriguing science discoveries of 2024
This year, our scientists illuminated the mysteries of memory and aging. They gained new insight into how cancer infiltrates the body and the three neurons that may be driving the impulse to eat. Across fields as diverse as neuroscience, CRISPR technology, and stem cell biology, these groundbreaking discoveries added up to another impressive year for advancement in the biomedical sciences. Here are some of the intriguing discoveries that came out of Rockefeller in 2024.
It turns out Vitamin A is playing an unexpected role in wound repair: hair follicle stem cells are involved in the healing process in a way mediated by retinoic acid, the active form of Vitamin A. A study from the laboratory of Elaine Fuchs found that, during this process, stem cells enter a temporary state of plasticity in which they adopt traits of multiple cell types. Given that an unchecked version of this kind of plasticity is present in some cancer stem cells, the findings raise the possibility that retinoids could also play a role in cancer suppression.
“This hasn’t been on the radar until now,” Fuchs says. “It’s an exciting front to now investigate.”
Robert B. Darnell’s lab unveiled a tool edging neuroscience much closer to understanding a phenomenon known as dendritic translation—a “holy grail for understanding memory formation,” he says. Dendritic translation is a coordinated burst of neuronal activity, which involves an uptick in localized protein production within the spiny branches that project off the neuron cell body and receive signals from other neurons at synapses. It’s a process key to memory—and its dysfunction is linked to intellectual disorders.
Using a new platform they developed, Darnell’s team identified several previously unknown regulatory mechanisms that drive dendritic translation. The work “defines a whole new biochemical pathway which fits with, complements, and vastly expands what we already knew about memory and learning,” Darnell says.
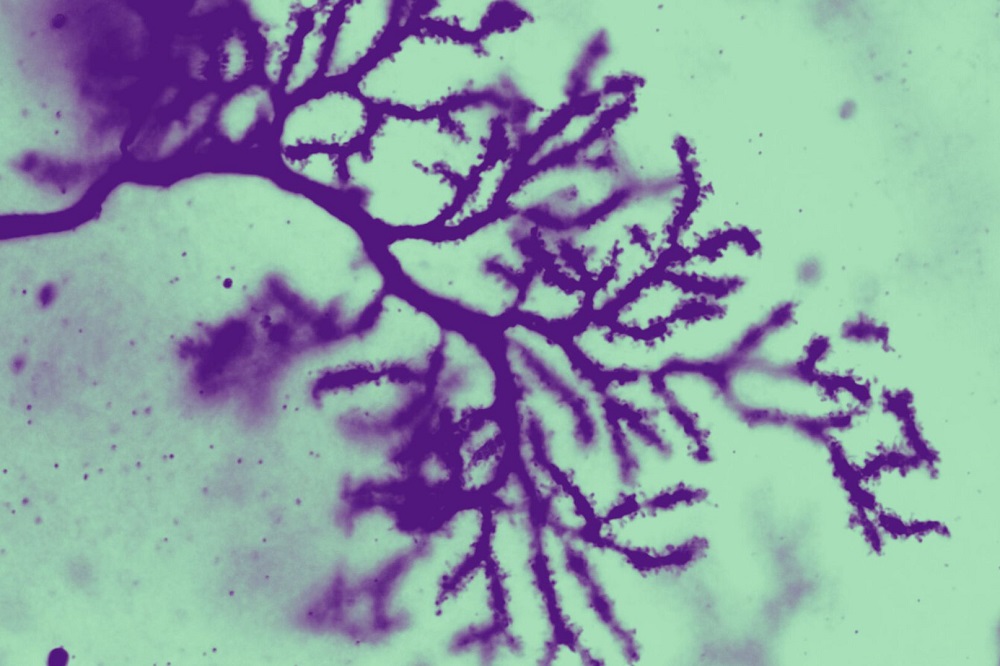
Purkinje cells in the cerebellum, stained and magnified 63 times, revealing fine details of the dendritic spines.
Autism spectrum disorder is tied to more than 70 genes, and the laboratory of Mary E. Hatten has long focused on the link between the ASTN2 gene and cerebellar changes in children with autism, as well as other neurodevelopmental conditions. This year, however, Hatten’s lab took that work to the next level with research demonstrating that knocking out ASTN2 in mice induces hallmark autism-like behaviors, including reduced vocalization, diminished social interaction, increased hyperactivity, and repetitive actions.
“It’s a big finding in the field of neuroscience,” Hatten says, and one that is inspiring her team to delve deeper into the hereditary components of autism. “We’re very excited that we’ve been able to show in detail what ASTN2 does, but there are a lot more genes to investigate.”
Metastasis’s surprising origins
A pair of compelling studies from the Tavazoie lab challenged conventional wisdom around cancer metastasis. Earlier this year, the lab demonstrated that sensory neurons play a direct role in promoting the growth and spread of breast cancer, providing the first ever evidence of peripheral nerves releasing a signal to enhance metastasis.
Most recently, Tavazoie’s team demonstrated, for the first time, that breast cancer metastasis may be, in part, a hereditary disorder driven by mutations in an inherited gene variant common to about 70 percent of white women. The scientists note their finding isn’t cause for alarm—the variant does not make people more likely to develop cancer, though it may up the risk for metastasis outcomes by between 2 to 22 percent—but it could open up a new pathway to treatment.
“We’ve been so focused on the cancer cells, the ‘seeds,’ that we’ve ignored the germline—the ‘soil’,” Tavazoie explains. “It’s now clear that focusing on the soil is critical.”
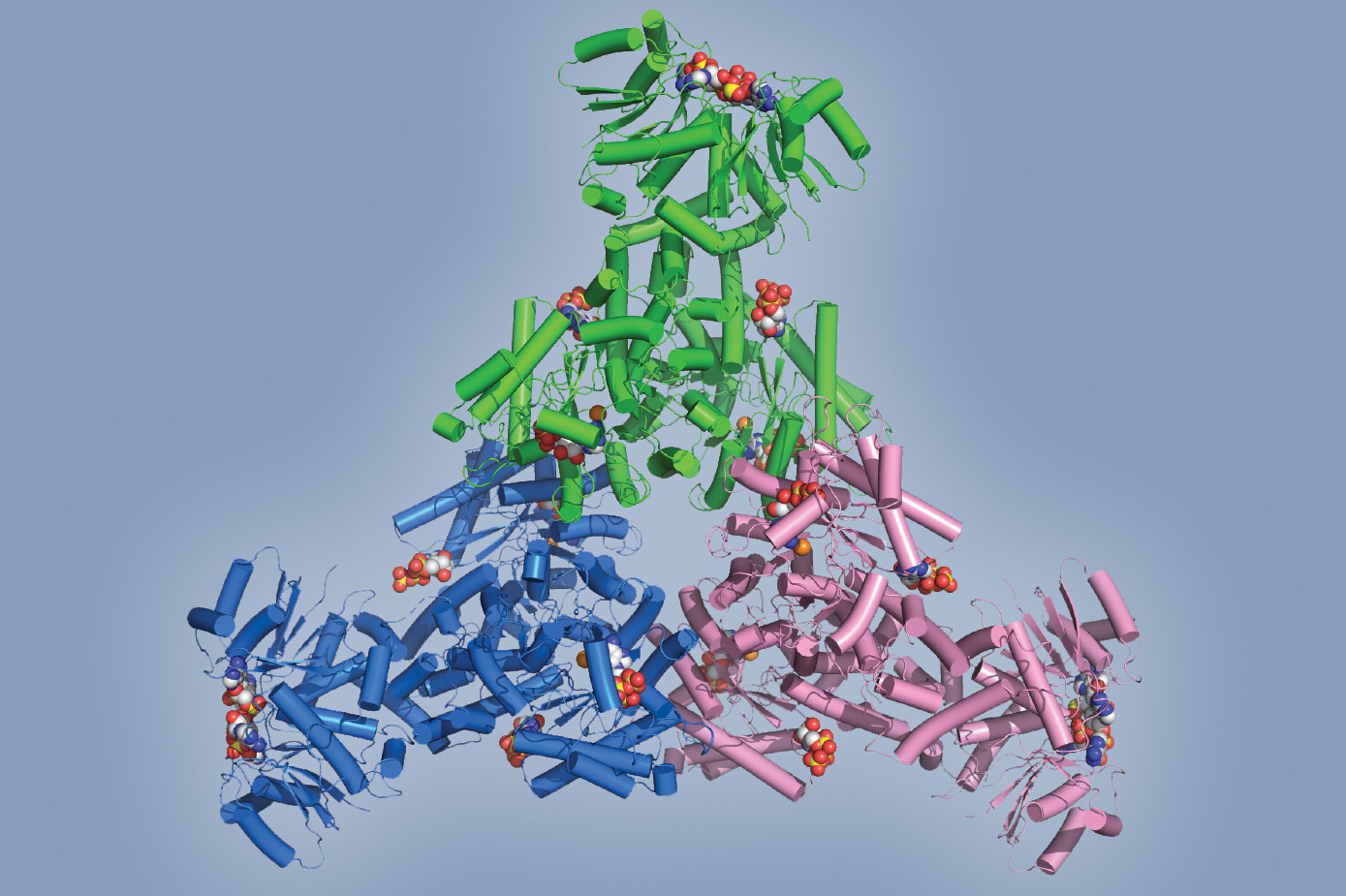
Multicolored model of a hexameric Cad1 protein
CRISPR-Cas9 has long been likened to a kind of genetic scissors, thanks to its ability to snip out any desired section of DNA with elegant precision. Now, thanks to work done in the lab of Luciano Marraffini, we know that certain CRISPR systems operate something like a molecular fumigator as well: In a collaboration with MSKCC, the team found a CRISPR system that also produces a flood of toxic molecules in response invading phages or plasmids, effectively shutting down the infected cell in order to prevent the contagion from spreading through the rest of the bacterial population.
“It’s a completely brand-new type of CRISPR chemistry,” says Christian Baca, a graduate student in the Marraffini lab. The group envisions one possible application of their discovery may be a diagnostic tool for infection—but it hints at much more. “It’s more evidence that CRISPR systems have an array of immune strategies at their disposal.”
Telomeres, the protective caps found at chromosome ends, must be kept at just the right length: too short, and dangerously accelerated aging ensues; too long, and they predispose their owners to cancer. Precise regulation is paramount, and Titia de Lange’s laboratory recently published work that fundamentally changes our understanding of telomere biology.
Among their findings, de Lange’s team discovered that two enzymes work in concert to ensure proper telomere maintenance. Scientists knew the telomerase enzyme helps prevent telomeres from growing shorter with each round of replication. But every DNA double helix has two strands to copy, and telomerase is capable of handling only one. Her team demonstrated how a second enzyme—the CST–Polα-primase complex—manages the other strand.
Related work elucidated a key mechanism for preventing telomerase from accidentally preserving damaged DNA: The main actor turned out to be the enzyme ATR kinase, which can stop telomerase from adding telomeres where it should not. de Lange is now exploring whether glitches in this self-protective process may help maintain the altered chromosomes that promote cancer progression.
‘Telomerase should only be acting at the natural ends of healthy chromosomes,” she says. “When it goes to work anywhere else, very bad things happen.”
Cell biology’s new mapping tool
Much progress has been made in the effort to catalog all our body’s different cell types, but the great mystery of how those cells work together to form tissues and organs remains unsolved. To do that, first scientists need a method of reliably tracking millions of intercellular interactions. Enter uLIPSTIC, a universal tool for tracking cell-to-cell interactions in vivo recently developed by the Victora lab.
The platform builds on an earlier version, LIPSTIC, which used a biochemical “kiss-and-run” mechanism to record fleeting interactions between immune cell types. By enabling any cell to tag any another during an interaction, the uLIPSTIC upgrade can, in principle, allow researchers to directly observe and quantify cellular networks in vivo.
“With uLIPSTIC we can ask how cells work together, how they communicate, and what messages they transfer,” Victora says.
Cancer cells are notorious for altering their metabolism to avoid immune detection, but research from Kivanç Birsoy’s lab revealed a surprising twist: certain lipids, once thought to be mere fuel for the tumor, actually act as fatty shields that help cancer cells evade the immune system. The study demonstrated that cancer cells specifically hoard these “glycosphingolipids” to obscure inflammatory signals and suppress immune responses.
The researchers used a combination of genetic screening and mouse models to pinpoint glycosphingolipids as essential to aggressive KRAS-driven pancreatic, lung, and colorectal cancers, and demonstrated that disrupting glycosphingolipid synthesis can make cancer cells more visible to the immune system. The findings suggest that targeting lipid metabolism, either through drugs or dietary interventions, could bolster the efficacy of existing immunotherapies.
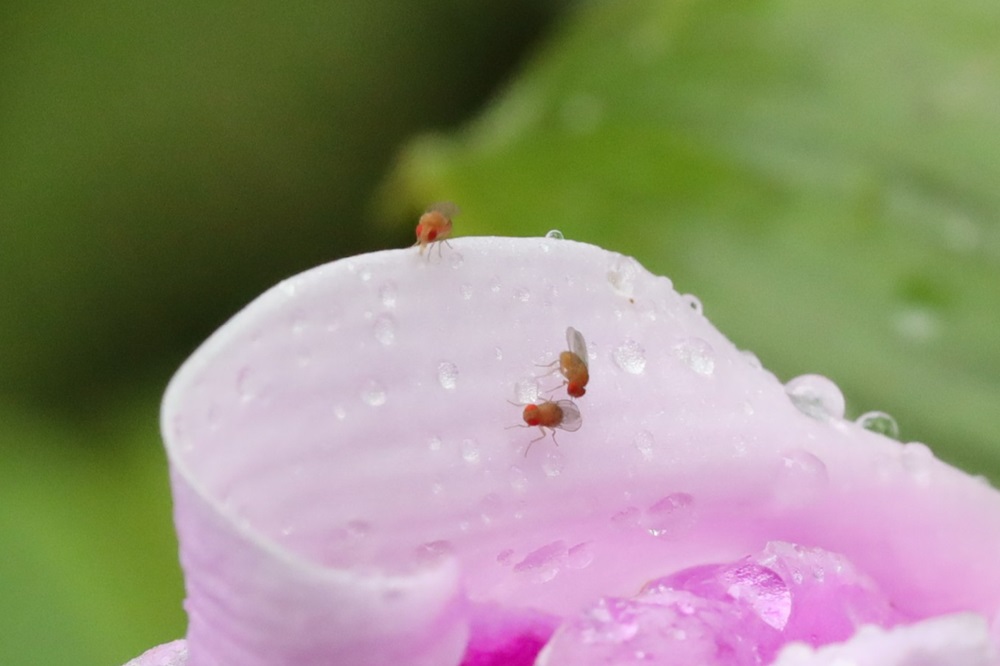
The mating behaviors of fruit flies, such as these Drosophila, offer scientists clues about the evolution of behavior.
Behavior’s evolutionary shortcut
Male fruit flies are masters of courtship, relying on pheromones in the dark and visual cues in the light to woo their mates. Research from Vanessa Ruta’s lab revealed how these tiny suitors efficiently evolve their ground game. The team found that fruit flies plug diverse sensory inputs, like novel pheromones, into conserved brain pathways. By repurposing these circuits rather than evolving entirely new ones, fruit flies can “plug and play”—adjusting mating rituals over relatively fast evolutionary time without needing to develop new neural pathways from scratch in response to new pheromones and environmental cues.
The study, which falls under the umbrella of Rockefeller’s Price Family Center for the Social Brain, also illustrates an experimental approach for studying how social behaviors evolve in general, beyond the realm of fruit fly brains. “We uncovered what we believe is a key neural mechanism that gives brain circuits the flexibility to rewire across species,” Ruta says.
Huntington’s most vulnerable cells
Huntington’s disease has long been linked to the expansion of tiny stretches of DNA bases known as CAG triplet repeats in the mutated Huntingtin gene, but this year, two studies from Nathaniel Heintz’s lab revealed some surprising insights into the mechanisms at play. The research demonstrated that CAG repeat instability and cell death are selective, affecting certain brain cell types while sparing others. As to why that may be, one key finding implicated elevated levels of certain DNA repair proteins, which exacerbate CAG expansion in medium spiny neurons, a particularly vulnerable cell type. Another discovery identified layer pyramidal neurons in the motor cortex as newly vulnerable: these cells exhibit unusually long CAG repeats and are prone to degeneration, potentially linking cortical and striatal dysfunction.
These results not only brought new understanding into the disease, but also new grounds for hope. “We need to understand these things in order to develop new treatments for this devastating disease,” Heintz says.
The decision to eat may come down to three neurons: Researchers in Jeffrey M. Friedman’s lab have identified a simple circuit in mice, comprised of a trio of brain cells, that serves as a lynchpin connecting hunger signals directly to jaw movement. The researchers found that activating these neurons suppressed appetite, while inhibiting them led to compulsive chewing—suggesting that the impulse to eat may be much more of a reflex-like response than previously thought.
“It’s surprising that these neurons are so keyed to motor control,” says study first author Christin Kosse, a research associate in the lab. “We didn’t expect that limiting physical jaw motion could act as a kind of appetite suppressant.”
Friedman and colleagues also found a new type of neuron that helps govern feeding—one that quickly counters hunger signals by promoting feelings of satiety. This work “added an important new component to the neural circuit that regulates appetite,” Friedman says. “It also solves a mystery about how feeding is regulated on different time scales by different neurons.” Together, the findings illuminate how the brain balances hunger and satiety, offering new avenues for tackling obesity and metabolic disorders.

A chart showing the explosive expansion in proportion of Granzyme K+ CD8+ T cells in mice across multiple organs as they age (Cao lab)
The laboratory of Junyue Cao has developed eight bespoke tools for single-cell sequencing to date, dramatically advancing a technology that’s already transforming how scientists understand disease states. Using one they dubbed EasySci, they recently were able to simultaneously scan more than 21 million cells from every major mouse organ across five stages of life.
Their findings reveal that certain cell populations change in every organ, both in the same way and at the same time, during specific stages of life. This suggests that aging is not a linear process but instead a developmental stage sparked by specific molecular cues.
“What’s more, some of these changes are controlled by the same molecular features,” says Cao. “So we might be able to target them to delay or even reprogram the aging process itself.”